Published by Bindi M. Doshi, PhD on Jun 10, 2024 10:04:00 AM
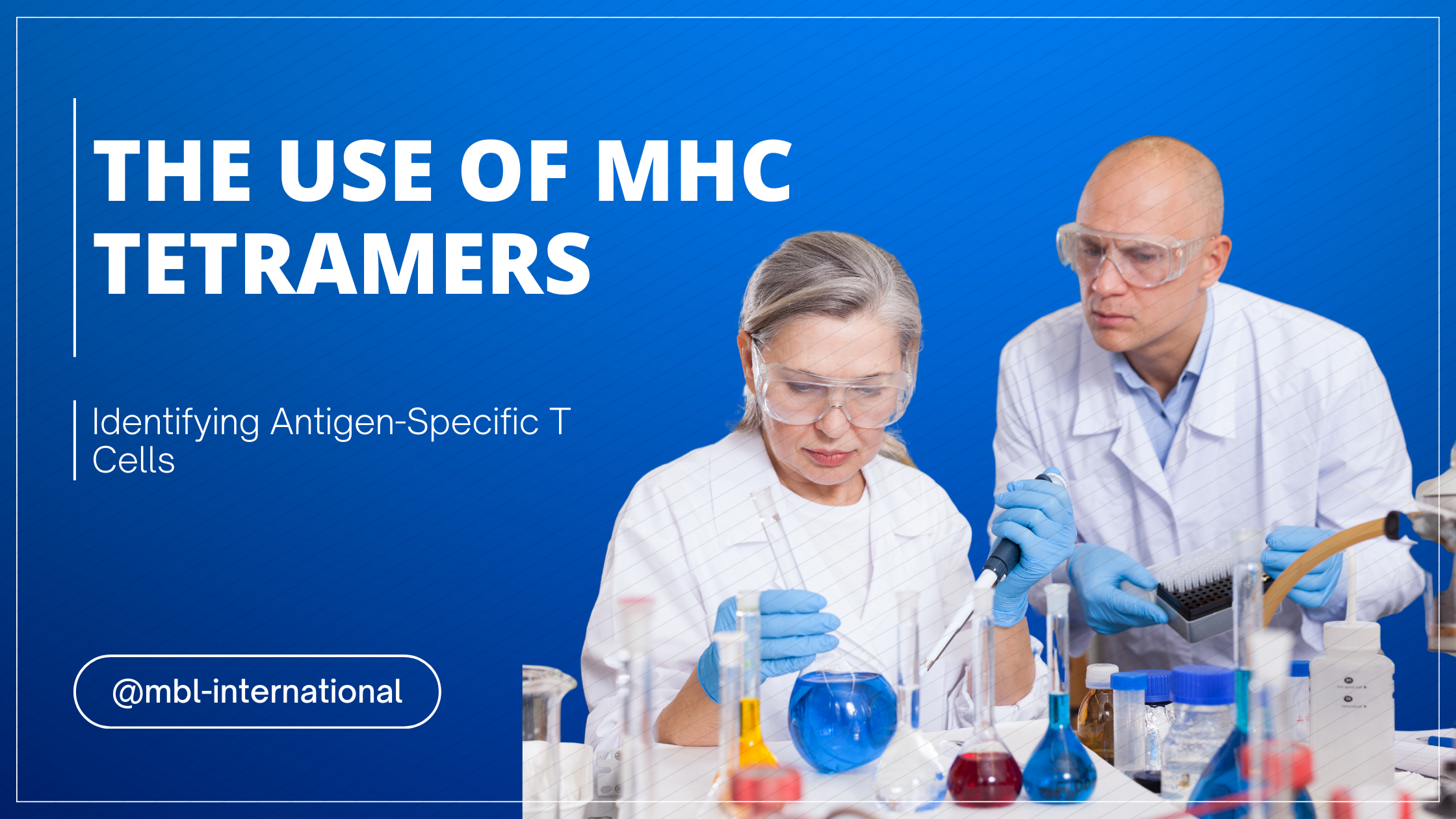
The immune system's ability to recognize and respond to specific pathogens or abnormal cells is crucial for maintaining health and combating diseases.
Central to this process are T cells, a type of white blood cell that plays a pivotal role in orchestrating immune responses.
Identifying and characterizing antigen-specific T cells has been a longstanding challenge in immunology, but it is critical for understanding immune function, developing vaccines, and treating immune-related disorders.
Major Histocompatibility Complex (MHC) and Antigen Presentation
T cells recognize antigens presented in the context of Major Histocompatibility Complex (MHC) molecules on the surface of antigen-presenting cells (APCs).
MHC molecules are highly polymorphic and can present a diverse array of peptide fragments derived from intracellular proteins.
This presentation allows T cells to distinguish between self and non-self antigens and mount appropriate immune responses.
Challenges in Identifying Antigen-Specific T Cells
Traditional methods for identifying antigen-specific T cells, such as ELISPOT and intracellular cytokine staining, have limitations in terms of sensitivity and specificity.
These methods often rely on functional assays that measure cytokine production or proliferation in response to antigen stimulation.
While informative, they do not directly identify T cells based on their antigen specificity.
Introduction to MHC Tetramers
MHC tetramers represent a significant advancement in the field of immunology by enabling direct visualization and quantification of antigen-specific T cells.
Tetramers are synthetic molecules composed of four MHC molecules loaded with a specific peptide antigen.
They are typically labeled with fluorochromes or other markers, allowing for their detection using flow cytometry.
Mechanism of Action
When introduced into a sample containing T cells, MHC tetramers bind specifically to T cell receptors (TCRs) expressed on the surface of T cells that recognize the cognate peptide-MHC complex.
This binding is highly specific, akin to a lock and key mechanism, and can be detected using flow cytometry.
By analyzing the fluorescent signal, researchers can quantify the frequency of antigen-specific T cells within a sample.
Applications in Research and Clinical Settings
The use of MHC tetramers has revolutionized our ability to study T-cell responses in various contexts:
- Vaccine Development: Assessing the immunogenicity of vaccine candidates by measuring antigen-specific T-cell responses.
- Infectious Diseases: Identifying and tracking pathogen-specific T cells during infection to understand immune evasion mechanisms and natural immunity.
- Autoimmune Diseases: Characterizing autoreactive T cells in autoimmune disorders to elucidate disease mechanisms and develop targeted therapies.
- Cancer Immunotherapy: Monitoring antigen-specific T cell responses in cancer patients treated with immunotherapies to evaluate treatment efficacy and predict clinical outcomes.
Advantages and Limitations
Advantages:
- High Specificity: Direct identification of antigen-specific T cells based on TCR binding.
- Quantitative Analysis: Enables precise quantification of rare antigen-specific T cell populations.
- Versatility: Applicable to a wide range of research and clinical settings.
Limitations:
- Cost and Complexity: Production and labeling of MHC tetramers can be resource-intensive.
- Heterogeneity: TCR binding affinity can vary among different T cell clones, affecting tetramer binding and detection efficiency.
Future Directions
Ongoing advancements in technology, such as multiplexing and combinatorial peptide-MHC libraries, hold promise for further enhancing the utility of MHC tetramers in immunological research.
These innovations facilitate the study of T cell repertoire dynamics, immune memory formation, and personalized medicine approaches.
Emerging Technologies and Methodological Advances
Recent developments in MHC tetramer technology have expanded its applications and improved its utility in immunological research. These include:
- Multimerization Techniques: In addition to traditional tetramers, advancements in multimerization technologies now allow the construction of pentamers, hexamers, or even higher-order complexes.
These multimers can enhance the sensitivity of T-cell detection by increasing the avidity of binding to TCRs.
- Peptide Exchange Strategies: Innovations in peptide exchange methodologies enable researchers to swap peptides bound to MHC molecules quickly.
This flexibility is advantageous for studying T-cell responses against multiple epitopes simultaneously or for investigating antigen cross-reactivity.
- Barcoding and Multiplexing: Barcoding approaches coupled with flow cytometry enable the simultaneous detection of multiple antigen-specific T-cell populations within a single sample.
This multiplexing capability streamlines experimental workflows and conserves precious biological samples.
- Single-Cell Analysis: Emerging single-cell technologies, such as single-cell RNA sequencing combined with tetramer staining, offer unprecedented insights into the heterogeneity of antigen-specific T-cell populations.
These techniques provide a deeper understanding of T cell differentiation, functional states, and lineage relationships.
Clinical Applications and Translational Impact
The clinical relevance of MHC tetramer technology extends beyond basic research, influencing diagnostic and therapeutic strategies:
- Monitoring Disease Progression: In chronic infections like HIV and hepatitis C, MHC tetramers facilitate longitudinal monitoring of antigen-specific T-cell responses.
This capability aids in assessing disease progression and evaluating the efficacy of antiviral treatments.
- Personalized Medicine: In the context of cancer immunotherapy, MHC tetramers play a pivotal role in identifying neoantigen-specific T cells.
These personalized antigens arise from tumor-specific mutations, making them ideal targets for immunotherapies aimed at enhancing anti-tumor immune responses.
- Transplantation Medicine: Pre-transplant screening using MHC tetramers allows for the detection of donor-specific T cells that could cause graft rejection.
This preemptive assessment informs donor selection and personalized immunosuppressive strategies post-transplantation.
Technological Challenges and Future Directions
Despite its significant advancements, MHC tetramer technology faces several challenges that warrant further exploration:
- Enhancing Sensitivity: Improving the detection limits of MHC tetramers to identify low-frequency antigen-specific T cells remains a priority.
Novel labeling strategies and sensitive detection platforms are actively being developed to address this issue.
- Incorporating Functional Assays: Integrating functional assays with tetramer staining offers comprehensive insights into T cell functionality beyond mere antigen specificity.
Combining tetramer technology with assays measuring cytokine secretion, cytotoxicity, or metabolic activity provides a more holistic view of T-cell responses.
- Understanding T Cell Exhaustion: Characterizing exhausted T cells, particularly in the context of chronic infections and cancer, presents a challenge due to their altered phenotype and functionality.
Leveraging MHC tetramer technology in conjunction with markers of exhaustion may elucidate mechanisms of T-cell dysfunction and inform therapeutic interventions.
Conclusion
MHC tetramers represent a cornerstone in immunological research, facilitating precise and quantitative analysis of antigen-specific T cells across diverse biological contexts.
As technological innovations continue to evolve and our understanding of T-cell biology deepens, the applications of MHC tetramer technology are poised to expand further.
By bridging the gap between fundamental research discoveries and clinical applications, MHC tetramers promise to revolutionize personalized medicine and therapeutic interventions, ultimately improving outcomes for patients affected by infectious diseases, autoimmune disorders, and cancer.
Visit MBL International Corporation for more information on MHC tetramers and their applications or to explore how they can advance your research or clinical practice.
FAQs
What are MHC tetramers, and how do they work?
MHC tetramers are synthetic molecules composed of four Major Histocompatibility Complex (MHC) molecules loaded with a specific peptide antigen. They are labeled with fluorochromes or other markers, enabling their detection using flow cytometry. When introduced into a sample containing T cells, MHC tetramers bind specifically to T cell receptors (TCRs) that recognize the cognate peptide-MHC complex. This binding allows for direct visualization and quantification of antigen-specific T cells.
What are the advantages of using MHC tetramers over traditional methods?
MHC tetramers offer several advantages:
- High Specificity: They directly identify antigen-specific T cells based on TCR binding.
- Quantitative Analysis: They enable precise quantification of rare antigen-specific T cell populations.
- Versatility: Applicable across various research and clinical settings, including vaccine development, infectious diseases, autoimmune disorders, and cancer immunotherapy.
What are the limitations of using MHC tetramers?
- Cost and Complexity: Production and labeling of MHC tetramers can be resource-intensive.
- Heterogeneity: TCR binding affinity may vary among different T cell clones, affecting tetramer binding and detection efficiency.
How are MHC tetramers used in research and clinical settings?
MHC tetramers are crucial in:
- Vaccine Development: Assessing vaccine immunogenicity by measuring antigen-specific T-cell responses.
- Infectious Diseases: Identifying and tracking pathogen-specific T cells during infections.
- Autoimmune Diseases: Characterizing autoreactive T cells to understand disease mechanisms.
- Cancer Immunotherapy: Monitoring antigen-specific T-cell responses to evaluate treatment efficacy.
What are the emerging technologies and advancements related to MHC tetramers?
Recent developments include:
- Multimerization Techniques: Beyond tetramers, advancements allow the construction of pentamers, hexamers, or higher-order complexes for increased sensitivity.
- Peptide Exchange Strategies: Facilitating the study of multiple epitopes simultaneously or investigating antigen cross-reactivity.
- Barcoding and Multiplexing: Simultaneous detection of multiple antigen-specific T cell populations within a single sample.
- Single-Cell Analysis: Providing insights into T cell heterogeneity and functional states.
How do MHC tetramers contribute to personalized medicine?
In cancer immunotherapy, MHC tetramers help identify neoantigen-specific T cells, which target tumor-specific mutations. They also aid in pre-transplant screening by detecting donor-specific T cells, informing personalized immunosuppressive strategies post-transplantation.
What are the challenges facing MHC tetramer technology moving forward?
Challenges include:
- We are enhancing Sensitivity: Improving detection limits to identify low-frequency antigen-specific T cells.
- Incorporating Functional Assays: Integrating tetramer staining with assays measuring cytokine secretion, cytotoxicity, or metabolic activity.
- Understanding T Cell Exhaustion: Characterizing exhausted T cells in chronic infections and cancer to inform therapeutic interventions.
What are the future directions for MHC tetramer technology?
Future advancements aim to enhance sensitivity, incorporate functional assays, and deepen understanding of T cell exhaustion. Ongoing innovations in labeling strategies and detection platforms will further expand the utility of MHC tetramers in immunological research and clinical applications.