Published by Bindi M. Doshi, PhD on Jun 17, 2024 5:01:00 AM
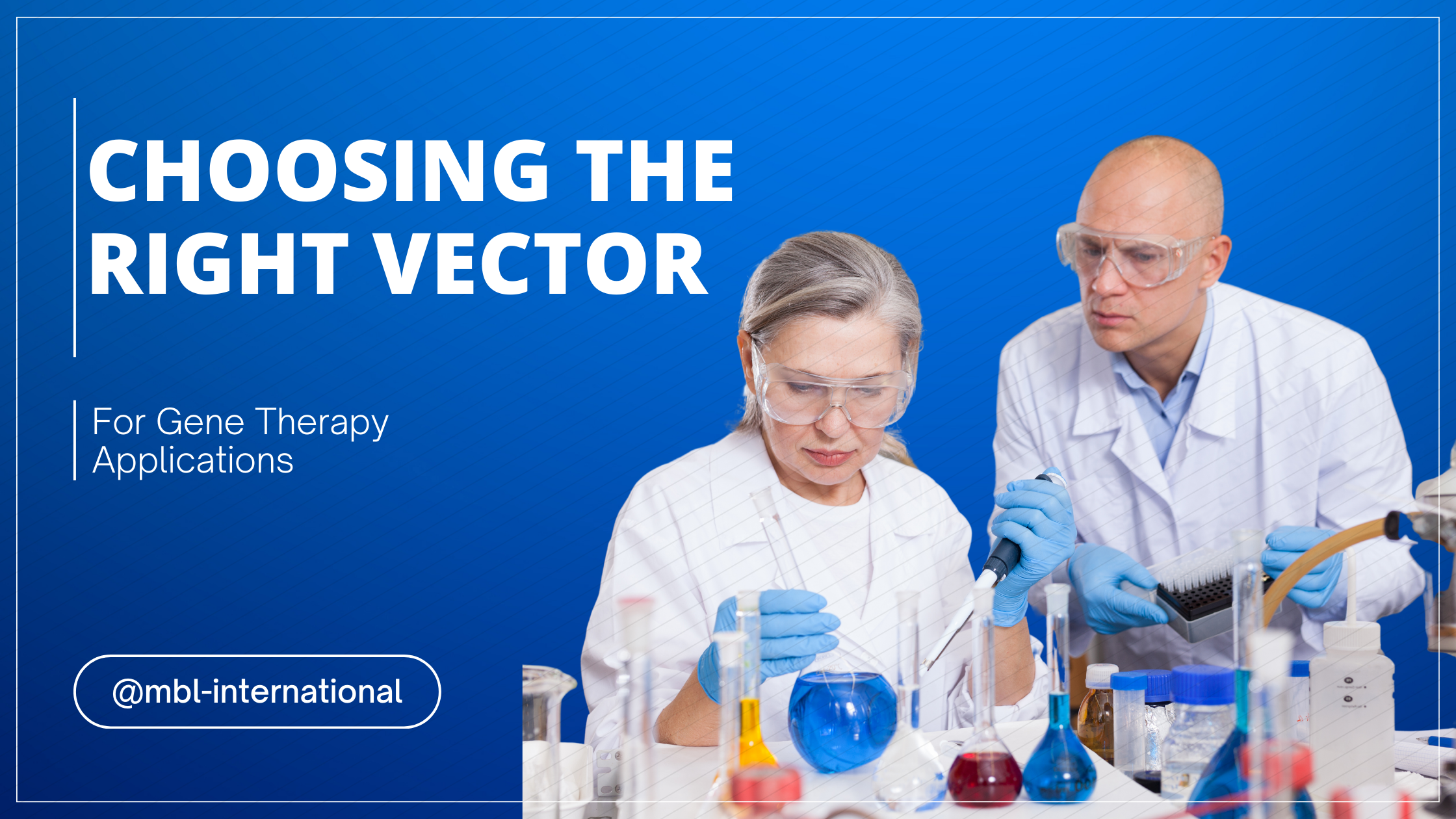
Gene therapy has emerged as a transformative approach in medicine, offering potential treatments for a broad spectrum of genetic disorders and diseases.
At the heart of this revolutionary field lies the critical decision of selecting an appropriate vector—a carrier that delivers therapeutic genes into target cells effectively and safely.
This article delves deeper into the considerations and advancements shaping the choice of vectors for gene therapy applications.
Understanding Vectors in Gene Therapy
Vectors, essential tools in gene therapy, act as vehicles to transport therapeutic genes into target cells.
These vectors can be broadly categorized into viral and non-viral systems, each offering distinct advantages and challenges.
Viral Vectors: Derived from naturally occurring viruses, these vectors have evolved mechanisms to infect cells and transfer genetic material efficiently.
Examples include retroviruses, lentiviruses, adenoviruses, and adeno-associated viruses (AAVs).
Viral vectors are preferred for their high transduction efficiency, ability to integrate into the host genome (in the case of retroviruses and lentiviruses), and capacity to deliver genes to both dividing and non-dividing cells.
Non-viral Vectors: These vectors encompass a range of synthetic or natural carriers that do not rely on viral components.
Examples include plasmid DNA, lipid-based nanoparticles, polymer-based carriers, and CRISPR-Cas systems.
Non-viral vectors are generally considered safer due to reduced immunogenicity and the absence of viral genome integration, though they often exhibit lower transduction efficiency compared to viral vectors.
Critical Considerations for Vector Selection
- Safety Profile: The safety of vectors is paramount in gene therapy.
Viral vectors must be carefully engineered to minimize the risk of causing disease or eliciting immune responses in patients.
Non-viral vectors typically pose lower risks of immunogenicity and adverse reactions but may induce inflammatory responses depending on the carrier and payload.
- Efficiency of Gene Delivery: The efficiency with which a vector can deliver genetic material to target cells determines its therapeutic efficacy.
Viral vectors excel in this aspect due to their natural ability to infect cells and their high transduction rates.
Non-viral vectors are continually improving, with advancements in nanoparticle technology and delivery methods enhancing their efficiency.
- Payload Capacity: The size of the genetic material (payload) that a vector can carry is crucial, particularly for delivering large or multiple therapeutic genes.
Viral vectors have size limitations imposed by their natural genome size, whereas non-viral vectors can accommodate larger DNA fragments, enabling more complex gene therapies.
- Target Cell Specificity: Tailoring vectors to target specific cell types or tissues enhances therapeutic precision and reduces off-target effects.
Viral vectors can be modified to display targeting ligands or utilize tissue-specific promoters.
Non-viral vectors are also being engineered to improve targeting specificity, although they generally exhibit less specificity compared to viral vectors.
- Scalability and Manufacturing: The scalability and cost-effectiveness of vector production are critical for translating gene therapies from research to clinical applications.
Viral vectors often require complex manufacturing processes and stringent quality controls, while non-viral vectors offer more straightforward production methods that may facilitate faster clinical translation.
- Immunogenicity and Host Response: Understanding how vectors interact with the immune system is essential for predicting and managing potential adverse reactions.
Viral vectors may induce immune responses due to viral proteins or capsid components.
In contrast, non-viral vectors typically provoke fewer immune reactions but may still trigger inflammatory responses depending on the delivery system used.
Current Trends and Future Directions
The field of gene therapy continues to evolve rapidly, driven by technological advancements and clinical successes. Current trends include:
- Enhanced Viral Vector Design: Improvements in viral vector engineering aim to improve safety profiles through capsid modifications and the development of self-inactivating vectors.
- Emerging Non-viral Technologies: Lipid nanoparticles, polymer-based carriers, and CRISPR-Cas systems are at the forefront of non-viral vector research, offering the potential for precise genome editing and improved delivery efficiency.
- Clinical Applications: Successful gene therapy treatments using AAV vectors for inherited retinal disorders, hemophilia, and muscular dystrophy highlight the growing clinical relevance and efficacy of gene therapies.
Advanced Viral Vector Engineering
Viral vectors are at the forefront of gene therapy due to their high efficiency in delivering therapeutic genes.
Recent advancements in viral vector engineering aim to enhance their safety, efficacy, and specificity:
- Capsid Modifications: The capsid, or outer protein coat, of viral vectors plays a crucial role in determining their infectivity and immune response.
Scientists are actively modifying viral capsids to improve their ability to evade immune detection, reduce toxicity, and enhance targeting of specific cell types or tissues.
For example, engineering mutations in the capsid proteins of AAV vectors have led to variants with altered tropism, enabling more precise targeting of desired cell types.
- Immune Evasion Strategies: Immune responses triggered by viral vectors can limit their therapeutic efficacy and pose safety concerns.
Researchers are developing strategies to evade immune detection, such as shielding viral vectors with polymers or peptides that mask immunogenic epitopes.
These modifications aim to prolong vector circulation in the bloodstream and reduce the likelihood of neutralizing antibody responses, thereby improving vector persistence and enhancing therapeutic outcomes.
- Self-Inactivating Vectors: Self-inactivating (SIN) vectors are designed to minimize the risk of insertional mutagenesis, a potential concern with integrating viral vectors.
SIN vectors contain deletions or mutations in essential viral genes required for replication after gene delivery.
This modification ensures that the therapeutic gene is expressed without the risk of reactivating viral replication, thereby enhancing safety profiles in clinical applications.
Emerging Non-viral Vector Technologies
While viral vectors dominate gene therapy due to their high transduction efficiency, non-viral vectors are gaining traction for their safety and versatility:
- Lipid Nanoparticles (LNPs): LNPs are emerging as promising non-viral delivery systems due to their ability to encapsulate and protect nucleic acids during delivery.
Advances in LNP formulation have improved their stability, biodistribution, and cellular uptake efficiency, making them suitable for delivering RNA-based therapies and genome editing tools like CRISPR-Cas9.
- Polymer-Based Carriers: Polymers offer a flexible platform for non-viral vector development, providing advantages such as tunable physicochemical properties, biocompatibility, and controlled release of therapeutic cargo.
Researchers are exploring novel polymer architectures and modifications to optimize gene delivery efficiency and minimize cytotoxicity.
- CRISPR-Cas Systems: The CRISPR-Cas gene editing technology has revolutionized the field of genome editing, offering precise control over genetic modifications.
Non-viral vectors are being engineered to deliver CRISPR-Cas systems into target cells, enabling precise genome editing for correcting genetic mutations underlying inherited diseases.
Future Directions and Challenges
The future of gene therapy vectors holds promise for advancing personalized medicine and treating a wide range of genetic disorders.
Key areas of focus include:
- Enhanced Targeting Strategies: Improving vector specificity for target tissues or cell types to minimize off-target effects and maximize therapeutic efficacy.
- Scalability and Manufacturing: Developing scalable production methods for both viral and non-viral vectors to meet clinical demand and reduce manufacturing costs.
- Regulatory Considerations: Addressing regulatory challenges to ensure the safety, efficacy, and quality of gene therapy vectors in clinical trials and commercial applications.
Conclusion
The field of gene therapy continues to evolve rapidly, driven by advancements in both viral and non-viral vector technologies.
These innovations are paving the way for personalized treatments and transformative therapies for previously incurable genetic diseases.
Whether through advanced viral vector engineering to enhance safety and specificity or the development of novel non-viral delivery systems like lipid nanoparticles and CRISPR-Cas systems, researchers are continually pushing the boundaries of what's possible in gene therapy.
Please visit MBL International Corporation to learn more about how we're contributing to the future of gene therapy through innovative solutions.
Discover how we're shaping the future of personalized medicine and exploring new frontiers in treating genetic disorders.
FAQs
What are vectors in the context of gene therapy?
Vectors are vehicles used to deliver therapeutic genes into target cells for gene therapy. They can be categorized into viral and non-viral systems, each with distinct properties and applications.
What are viral vectors, and how do they work?
Viral vectors are derived from viruses and are engineered to deliver genetic material into cells efficiently. Examples include retroviruses, lentiviruses, adenoviruses, and adeno-associated viruses (AAVs). They are preferred for high transduction efficiency and capability to integrate into the host genome in some instances.
What are non-viral vectors, and what advantages do they offer?
Non-viral vectors encompass synthetic or natural carriers that do not rely on viral components. Examples include plasmid DNA, lipid-based nanoparticles, polymer-based carriers, and CRISPR-Cas systems. They are considered safer due to lower immunogenicity and lack of viral genome integration, though they may have lower transduction efficiency.
How do I choose between viral and non-viral vectors for gene therapy?
The choice depends on factors such as safety profile, efficiency of gene delivery, payload capacity, target cell specificity, scalability in manufacturing, and immunogenicity. Viral vectors offer high efficiency but may pose safety risks, while non-viral vectors are safer but less efficient in delivery.
What safety considerations should I keep in mind when selecting a vector?
Safety is crucial in gene therapy. Viral vectors require careful engineering to minimize risks of immune responses or adverse effects. Non-viral vectors typically have lower immunogenicity but may induce inflammatory responses depending on the carrier used.
How does the efficiency of gene delivery differ between viral and non-viral vectors?
Viral vectors generally have higher transduction rates due to their natural ability to infect cells. Non-viral vectors are improving with advancements in nanoparticle technology but often have lower delivery efficiency compared to viral vectors.
Can vectors be tailored to target specific cell types or tissues?
Yes, both viral and non-viral vectors can be engineered to enhance targeting specificity. Viral vectors can be modified to display targeting ligands or use tissue-specific promoters, and non-viral vectors are also being developed for improved targeting capabilities.